UV light, cancer and more
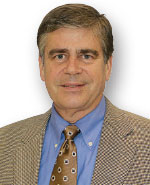
Karl Danneberger, Ph.D.
Sunlight is made up of the visible light spectra, which is comprised of wavelengths between 380 nm and 700nm. Bookending the visible light spectra is ultraviolet (UV) and infrared wavelengths. Ultraviolet wavelengths are associated with skin tanning and cancer in humans.
While part of the light spectrum, ultraviolet is invisible to the naked eye. Ultraviolet radiation is broken down by wavelength into three main groups, UV-A, UV-B and UV-C. UV-A is the longest of the three wavelengths at 320 nm to 400 nm and is closest to the visible light spectrum. Ninety-five percent of UV radiation is UV-A. UV-A penetrates deeper into the skin than UV-B. Tanning, skin aging and wrinkling are associated with UV-A. Until recently, however, scientists didn’t associate UV-A with damage to epidermis cells (outermost cells), which are the cells most associated with skin cancer.
UV-B causes sunburn. This wavelength damages primarily the human skin’s epidermal, or top layer. It’s this damage in the epidermal layer that leads to skin aging and skin cancer. Generally, the greatest amount of UV-B occurs from April to October between the hours of 10 a.m. to 4 p.m. However, UV-B occurs year round.
UV-C is the shortest of the ultraviolet wavelengths (less than 290 nm). UV-C receives little attention compared to UV-A and UV-B because these short wavelengths are absorbed by the ozone layer and fail to penetrate to the earth’s surface, so the impact of natural UV-C on Earth’s biological systems (either humans or turf) is non-existent. However, artificially produced UV-C around 254 nm has been used for more than a century as a sterilizing tool for microbial contamination. With the increased resistance to antibiotics in hospitals, UV-C lamps are being increasingly used as germicidal/disinfectant treatments for microbes. UV-C is not known to cause cancer in these situations, probably because of its lack of penetration through dead cells on the skin surface.
UV-C has been evaluated and tested in plants as a possible disease-controlling agent. It has been used in small, controlled environments like greenhouses and in post-harvest situations with fruits and vegetables. UV-C is highly absorbed by organic substances like DNA, which can result in severe damage to microbes. In general, UV-C is absorbed by the cell proteins and DNA, causing damage through metabolic disruption, while the DNA is altered so the organism can no longer replicate.
Interestingly, UV-C radiation is associated with physiological changes in plants at low doses. In some studies on fruits and vegetables, treatment post-harvest resulted in greater stress resistance. In the widely used research plant Arabidospsis, UV-C induced damage in the plant resulted in greater plant resistance to downy mildew. To induce better stress resistance, low doses, called hormic doses, of UV-C are applied in short pulses.
So what about using UV-C for disease control in turf? Most golf courses aren’t small, contained units like a greenhouse or storage facility, so the idea of a UV-C machine traveling across a golf course doesn’t sound practical or financially feasible, except perhaps for specific green use. However, UV-C devices for controlling diseases on athletic fields are being proposed and tested.
Would such devices work? Research shows the potential for directly killing microbes through mutations to plants, making them resistant to pathogens. I suspect the potential for disease control in turf is feasible.
Now that UV-C has been used on annual crops in greenhouses and in post-harvest situations, what are its long-term effects on perennial turf? Can mutations induced to enhance disease resistance also be detrimental to plants? How intense should be the doses and frequency? In the absence of UV-C DNA damage, can repair occur, or will continued exposure at high UV-C intensity result in detrimental effects?
Given all this and with interest growing, science will have to tell us the positive and negative impacts of UV-C on turf.